Pressure Swing Adsorption (PSA) oxygen enrichment technology is widely used in medical, industrial, and environmental protection fields due to its simple operation, low energy consumption, and compact equipment. However, there remains some ambiguity regarding whether PSA oxygen enrichment increases oxygen content, boosts oxygen partial pressure, or achieves both. Clarifying this is crucial for accurately understanding its application characteristics and optimizing its use.
PSA oxygen enrichment technology separates oxygen and nitrogen from air based on the principle of pressure swing adsorption, primarily by increasing oxygen concentration. In certain cases, it can also lead to an increase in oxygen partial pressure. Therefore, depending on the application scenario and requirements, PSA oxygen enrichment technology can increase oxygen content and, under specific conditions, enhance oxygen partial pressure.
1. Basic Principles of PSA Oxygen Enrichment Technology
1.1 Adsorption Separation Mechanism
PSA technology relies on the selective adsorption of different gases by adsorbents under varying pressures. In PSA oxygen enrichment systems, adsorbents such as zeolite molecular sieves are commonly used, as they have a higher affinity for nitrogen than oxygen. During the high-pressure adsorption phase, the feed gas (e.g., air) enters the adsorption tower, where nitrogen is preferentially adsorbed by the adsorbent. The remaining gas, with a higher oxygen content, exits as oxygen-enriched gas. Once the adsorbent reaches saturation, the system enters the low-pressure desorption phase, where the pressure is reduced to release the adsorbed nitrogen, regenerating the adsorbent for the next adsorption cycle.
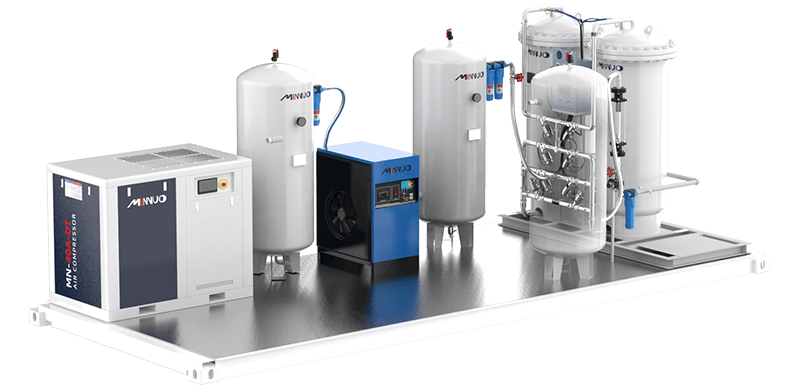
1.2 Equipment Operation Process
A typical PSA oxygen enrichment system consists of multiple adsorption towers. Through a well-designed control system, the towers alternate between adsorption, pressure equalization, and desorption phases. For example, in a two-tower PSA system, while one tower is in the adsorption phase producing oxygen-enriched gas, the other undergoes desorption and regeneration. Pressure equalization steps recover some of the gas pressure, improving system efficiency. This multi-tower cyclic operation ensures a continuous and stable supply of oxygen-enriched gas while enhancing adsorbent utilization and reducing energy consumption.
2. Basic Concepts of Oxygen Content and Oxygen Partial Pressure
2.1 Oxygen Content
Oxygen content refers to the proportion of oxygen in a gas mixture, typically expressed as a volume or mole fraction. Under standard conditions, the oxygen volume fraction in air is approximately 21%. Accurate measurement of oxygen content is essential for assessing gas quality and suitability. Common measurement methods include electrochemical, paramagnetic, and gas chromatography techniques. Electrochemical methods, based on the electrochemical reaction between oxygen and electrodes, offer fast response and low cost, making them suitable for real-time monitoring. Paramagnetic methods, utilizing oxygen’s paramagnetic properties, provide high precision and are ideal for applications requiring accurate oxygen content measurement. Gas chromatography, capable of precise separation and quantitative analysis of gas mixtures, is often used in research and high-precision testing.
2.2 Oxygen Partial Pressure
According to Dalton’s Law of Partial Pressures, the partial pressure of a gas component in a mixture equals its mole fraction multiplied by the total pressure. At standard atmospheric pressure (101.325 kPa), the oxygen partial pressure in air is approximately 21.278 kPa (101.325 kPa × 21%). Oxygen partial pressure is significant in various fields. For instance, in physiology, oxygen uptake and metabolism in human tissues depend on oxygen partial pressure. In industrial combustion processes, oxygen partial pressure directly affects the rate and completeness of combustion reactions.
3. Impact of PSA Oxygen Enrichment on Oxygen Content and Partial Pressure
3.1 Increase in Oxygen Content
PSA oxygen enrichment technology can significantly increase the oxygen content in mixed gases. By optimizing the performance of adsorbents, the structure of adsorption towers, and operational parameters, the oxygen concentration in air can be raised from 21% to 30%–90% or even higher. In the healthcare field, small PSA oxygen enrichment devices typically raise the oxygen content to 30%–40%, meeting the needs of patients requiring mild oxygen supplementation. A clinical study on patients with chronic obstructive pulmonary disease (COPD) showed that using oxygen-enriched gas with an oxygen concentration of 30%–35% for adjunctive therapy can effectively improve respiratory function and quality of life. In the industrial sector, large PSA oxygen enrichment units can raise the oxygen concentration to over 90% for processes such as steelmaking and glass manufacturing, thereby improving production efficiency and product quality.
Adsorbent performance is a key factor among several elements that influence the effectiveness of oxygen content enhancement. Novel adsorbents like metal-organic frameworks (MOFs), with their ultra-high surface areas and tunable pore structures, exhibit higher adsorption selectivity and capacity than traditional zeolite molecular sieves, potentially further improving oxygen enrichment efficiency. Adsorption tower design is also critical. Proper internal components, such as efficient gas distributors and structured packing, ensure uniform gas distribution within the tower, enhancing mass transfer and oxygen content. Additionally, optimizing operational parameters like gas flow rate, adsorption, and desorption times can significantly impact oxygen content enhancement.
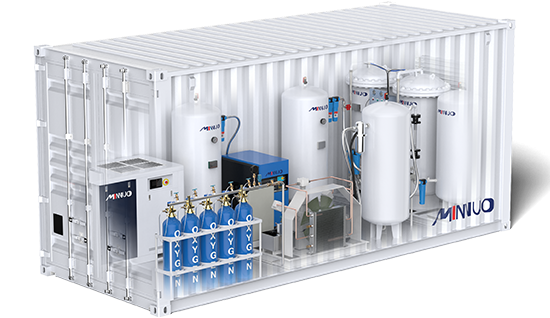
3.2 Increase in Oxygen Partial Pressure
As oxygen content increases, oxygen partial pressure naturally rises if the total pressure remains constant or is appropriately adjusted. In a closed system with constant atmospheric pressure, increasing oxygen content from 21% to 40% raises oxygen partial pressure from 21.278 kPa to 40.53 kPa (101.325 kPa × 40%). In industrial applications, to meet high oxygen partial pressure requirements, the output gas from PSA oxygen enrichment systems is often pressurized. For example, in glass furnaces, pressurizing oxygen-enriched gas to 0.3-0.5 MPa significantly increases oxygen partial pressure, enhancing combustion efficiency and reducing fuel consumption by 15%-25%.
The choice and control of pressurization methods are critical in practical applications. Centrifugal and screw compressors are commonly used for pressurization. Centrifugal compressors, which are suitable for high – flow, low – to – medium pressure applications, offer wide flow regulation and stable operation. As for screw compressors, they excel in medium – to – high pressure, low – to – medium flow scenarios and feature compact structures and easy maintenance. When selecting pressurization methods and levels, factors such as process requirements, equipment costs, energy consumption, and operational stability must be considered. Excessive pressurization can increase equipment investment and operating costs, potentially leading to unstable operation and affecting production continuity.
4. Considerations in Practical Applications
4.1 Healthcare Sector
In the healthcare field, the main focus is on maintaining an appropriate oxygen concentration to meet the physiological needs of patients. For most patients requiring oxygen therapy, an oxygen concentration of 30% to 40%, combined with normal respiratory pressure (about 101.325 kPa), provides effective supplementary treatment. Excessively high oxygen concentrations may lead to adverse reactions such as oxygen toxicity, so precise control of oxygen levels is crucial. Home-use PSA oxygen concentrators, with their simple user interface and automatic control systems, can deliver stable low-concentration oxygen-enriched air to patients, improving symptoms of hypoxia and enhancing their quality of life.
4.2 Industrial Combustion Sector
In industrial combustion processes, such as glass manufacturing and steel smelting, increasing oxygen partial pressure significantly enhances combustion efficiency. Glass furnaces using oxygen-enriched combustion technology not only improve glass melting speed and quality but also reduce emissions of pollutants like nitrogen oxides (NOx). Studies show that using oxygen-enriched air with 27%-30% oxygen content, pressurized to 0.3-0.4 MPa, in glass furnaces can reduce fuel consumption by 20%-30% and NOx emissions by 40%-60%. In steel smelting, oxygen-enriched blast technology accelerates iron ore reduction reactions, improving blast furnace efficiency and reducing coke ratios.
4.3 Aquaculture Sector
In aquaculture, PSA oxygen enrichment technology effectively increases dissolved oxygen levels in water. By introducing oxygen-enriched air into aquaculture water, oxygen partial pressure is increased, promoting the growth and reproduction of aquatic organisms and reducing disease incidence. An experiment on shrimp farming showed that using PSA oxygen enrichment devices to increase dissolved oxygen levels from 6-7 mg/L to 8-9 mg/L improved shrimp growth rates by 15%-20% and survival rates by 10%-15%. Adequate dissolved oxygen supports respiratory metabolism in aquatic organisms, enhancing immunity and feed utilization.
4.4 Chemical Synthesis Sector
In chemical synthesis, certain reactions require specific oxygen partial pressures. For example, in ethylene oxide synthesis, precise control of oxygen content and partial pressure is crucial for improving reaction conversion rates and selectivity. PSA oxygen enrichment technology allows flexible adjustment of oxygen content and partial pressure based on reaction requirements, optimizing reaction conditions. By maintaining appropriate oxygen partial pressure and reaction temperature, ethylene oxide selectivity can be increased to 85%-90%, significantly improving production efficiency and product quality.
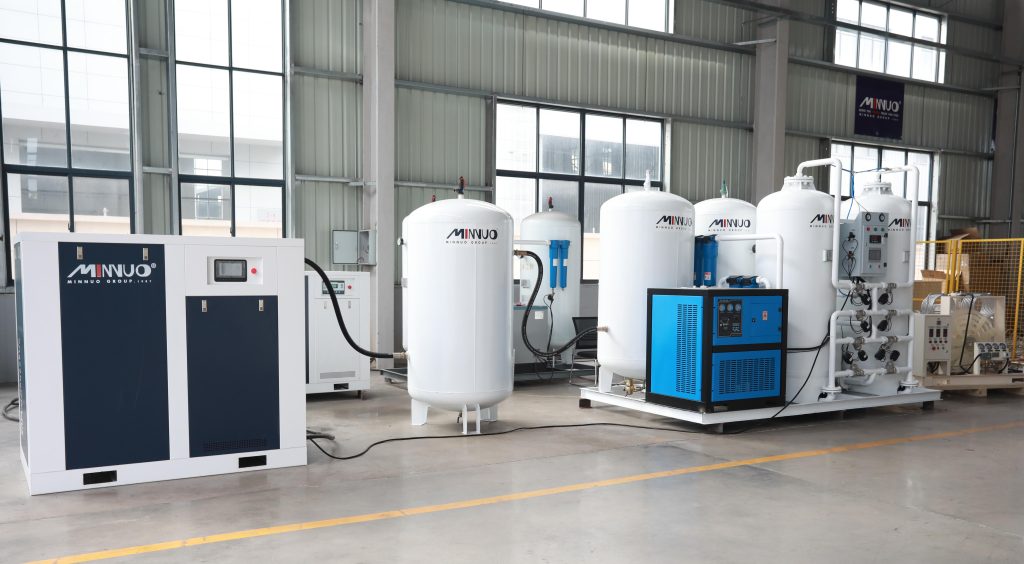
5. Future Development Trends
5.1 Adsorbent Development
Future research on adsorbents will focus on improving adsorption capacity, selectivity, and lifespan. The exploration and development of novel adsorbent materials, such as nanotechnology-based adsorbents, are key research areas. By precisely controlling nanoscale pore structures and surface properties, these materials promise more efficient adsorption of nitrogen and other impurities, further enhancing oxygen enrichment efficiency. Additionally, developing adsorbents with high stability and resistance to poisoning will extend their lifespan, reduce replacement costs, and improve the long-term operational stability of PSA oxygen enrichment systems.
5.2 Intelligent Equipment Control
With the rapid advancement of artificial intelligence and automation technologies, intelligent control of PSA oxygen enrichment equipment will become a significant future trend. Advanced sensors will be installed to monitor real-time operational parameters such as gas flow, pressure, temperature, and oxygen content. Intelligent algorithms will analyze and process this data, enabling precise equipment control. Intelligent control systems can automatically optimize adsorption, desorption, and other operational parameters based on different process requirements and feed gas compositions, improving equipment adaptability and operational efficiency while reducing energy consumption and labor costs.
5.3 Integration with Other Technologies
The integration of PSA oxygen enrichment technology with other gas separation or chemical reaction processes is another future trend. Combining PSA with membrane separation technology leverages the efficiency of membrane separation and the flexibility of PSA, enabling finer separation of gas mixtures and producing higher-purity oxygen-enriched gas. In some chemical reactions, integrating PSA oxygen enrichment systems with reaction devices for in-situ oxygen enrichment can enhance reaction rates and conversion rates, simplify processes, and reduce equipment investment and operating costs.
6. Conclusion
In summary, PSA oxygen enrichment technology not only increases oxygen content in gas mixtures but also effectively enhances oxygen partial pressure under appropriate operating conditions. By precisely regulating oxygen content and partial pressure according to specific application needs, the advantages of PSA oxygen enrichment technology can be fully utilized, achieving efficient, energy-saving, and environmentally friendly goals.
As a manufacturer with over 30 years of experience in oxygen generator development, MINNUO welcomes inquiries from those interested in oxygen enrichment equipment!